Active site seeking
Heterogeneous catalytic materials comprise a variety of sites with different intrinsic activity. Due to the complexity of most catalysts, discovering the structural motive governing catalytic activity and selectivity is of importance both to obtain fundamental understanding as well as to design improved catalysts with better performance. In my research, I have developed single-atom catalysts ideally suited for the investigation of catalytic active sites. This knowledge was then used to design improved catalysts for diverse reactions (such as the CO or alcohol oxidation reactions and the hydrodeoxygenation reaction). This unique catalyst system is also suitable to investigate fundamental processes such as the hydrogen spillover and its relation to hydrogenation catalysis. Beyond understanding and designing active site structures, I developed interest in promoting heterogeneous catalysis through external stimuli (e.g. electric potentials or magnetic fields), both statically and dynamically.
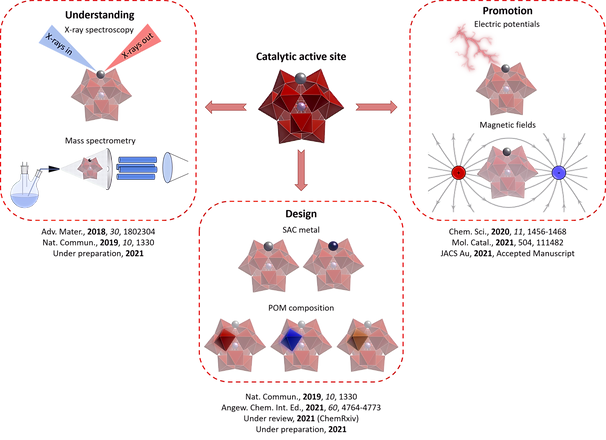
Understanding active sites
The investigation of catalytic active sites usually is hindered by the complexity of the material – usually oxide-supported metallic nanoparticles – as well as inherent limitations of techniques to probe solid materials with high spatial and temporal resolution under reaction conditions. We circumvent the first problem with the design of single-atom catalysts supported on polyoxometalates, molecularly defined metal-oxo clusters. Those polyoxometalates essentially only have one strong adsorption site for metal atoms and thus, all active sites can be assumed to be structurally identical. This material offers opportunities for in situ spectroscopic approaches (e.g. XAS, XPS, IR, etc.) as demonstrated for the CO oxidation reaction (Nat. Commun., 2019, 10, 1330). With this catalyst system, we went one step further and borrowed methodology from mechanistic analysis of homogeneous catalysis, in particular electrospray ionization mass spectrometry. Polyoxometalates can serve as a catalyst support in the solid state but also in the liquid phase where polyoxometalates function as ligands rendering the single-atom catalyst homogeneous. After establishing this methodology for different oxidation reactions (Angew. Chem. Int. Ed., 2021, 60, 4764-4773), we were able to extend this approach to studying the hydrogen spillover behavior and how spilled-over H contributes to catalysis.

Designing active sites
Besides serving as an ideal platform to understand reaction mechanisms, the composition and thus properties of polyoxometalates can be easily tuned. For the CO oxidation reaction, we for example found that the support reoxidation is the rate-determining step and were thus able to improve the catalytic activity significantly by decreasing the oxidation potential (i.e. how resistant the support is to re-oxidation). When the metal-support interface is carefully chosen, the stabilization of zero valent Pd atoms becomes possible opening new avenues to modify the reactivity of single-atom catalysts. We demonstrated this improved activity for the hydrodeoxygenation of various biomass-derived oxygen-containing substrates with unprecedented activity at low temperature (e.g. the deoxygenation of 5-HMF to 2,5-DMF with high reaction rates at below room temperature; ChemRxiv).

Promoting active sites
Despite the indisputable success of conventional synthesis-based approaches to manipulate the performance of heterogeneous catalysts, future research on catalysis engineering will likely go beyond the catalyst itself. Several auxiliary promotion methods, either promoting the reactivity of reagents or enabling optimized adsorbate–catalyst interactions, have been proven as viable strategies to enhance catalytic reactions. Those auxiliary promotion methods range from electric/magnetic fields and electric potentials to mechanic stress, significantly altering the properties of reagent molecules and/or the surface characteristics of nanostructured catalysts (Chem. Sci., 2020, 11, 1456-1468). Apart from static enhancement effects, they in principle also allow for spatially and temporally variable modifications of catalyst surfaces. While few of those methods have been demonstrated (Mol. Catal., 2021, 504, 111482), most are only theoretically predicted, opening exciting avenues for future research. For instance, we were able to show recently that the non-Faradaic ethylene hydrogenation reaction can be improved by a factor of 5 by applying dynamic potentials on catalytic metal surfaces (JACS Au, 2021, Accepted Manuscript).
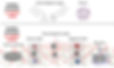